TRANSLATE consortium member and Work Package 1 leader, Professor Steffen Hardt (Technische Universität Darmstadt), tells us about the physics of thermoelectric energy conversion in nanochannels.
Solid-state materials that can convert thermal energy into electric energy have been known for a long time. These thermoelectric materials are based on the Seebeck effect and have so far only found applications in quite specific areas. This is partly due to their low energy conversion efficiencies, but also because the materials involved are expensive and not well suited for large-scale usage. In the past few years, thermoelectric energy conversion based on nanopores or nanochannels filled with an electrolyte solution has emerged as a new paradigm. The underlying physics is only beginning to be understood, and in the following paragraphs, some of the key phenomena involved will be explained.
One key phenomenon is the existence of an electric double layer (EDL) at the nanochannel walls. In its simplest form, the EDL consists of electric charges attached to the channel walls and a diffuse layer of mobile charges, which are ions contained in the electrolyte solution. This diffuse layer may have a thickness of the order of 10 nanometers. The ions are attracted to the walls by their counter charges, but they also experience collisions with the surrounding water molecules. As a result, the attractive force is exactly compensated by the tendency of the ions to diffuse away from the walls, and a stationary ion cloud forms.
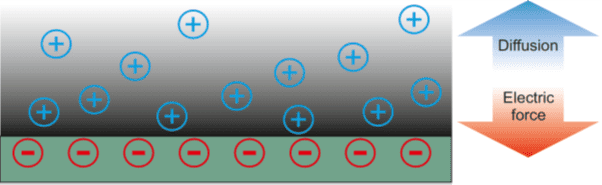
This equilibrium configuration of the EDL is disturbed when the temperatures in different sections of a nanochannel are different. In a channel with a hot and a cold end, the EDL usually expands at the hot end and shrinks at the cold end. As a consequence, the ion cloud is no longer in equilibrium, which means that the tendency of the ions to diffuse away from the walls is no longer fully compensated by electrostatic attraction. Instead, concentration gradients form along the channel, which induce ion migration. As a result, a voltage builds up along the channel, indicating that a part of the thermal energy has been converted into electric energy.
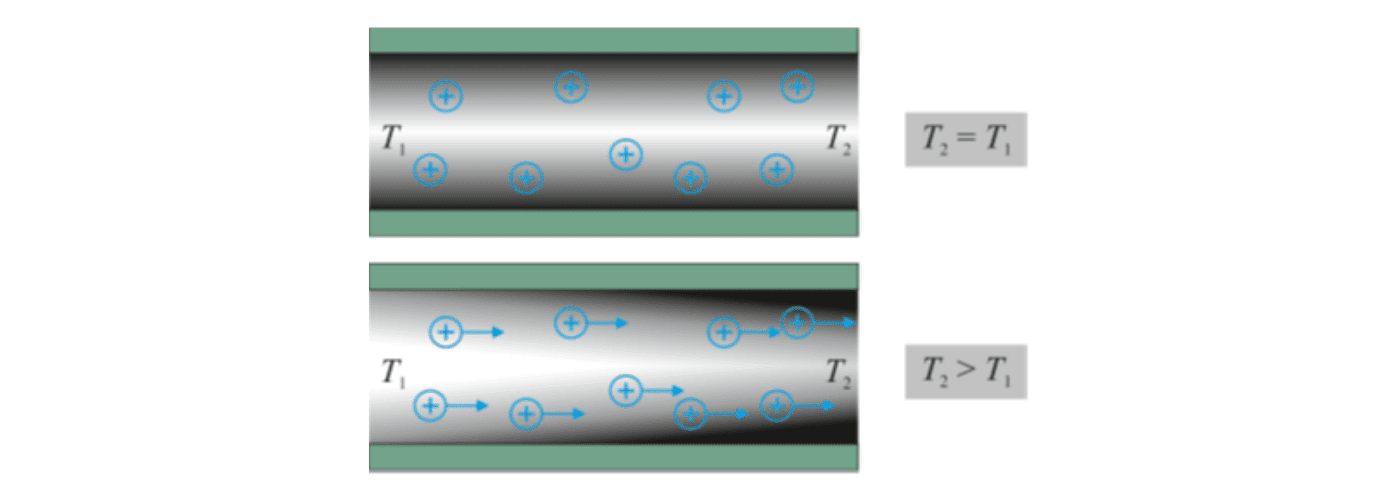
Within the TRANSLATE project, the goal is to determine nanochannel configurations that result in as-large-as-possible conversion efficiencies. For this purpose, coupled numerical simulations of the flow, the electric field and the ion concentration fields inside a channel are being conducted. The strength of the ion flux that finally creates the thermovoltage depends on a superposition of three different effects. Firstly, there is diffusive ion motion due to gradients in the ion concentration field. Secondly, in an electric field, ions migrate relative to the background fluid. Last but not least, usually there will be a fluid flow inside the nanochannel, which results in advective ion transport.
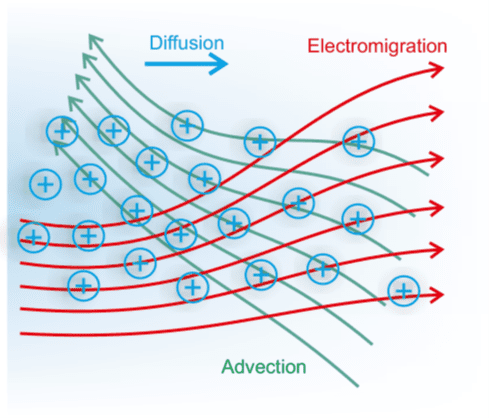
Read more: Nanochannel Theory Explained